ABSTRACT
Since 2006, a swath of north-central Oklahoma has been plagued with a series of small earthquakes, most having magnitude less than 4.0. They are generally believed to be associated with wastewater injection associated with oil and gas wells. The increased quantity of small-magnitude earthquakes poses a problem for a small but important group. These events have been concentrated in a rectangular area that includes Norman and Stillwater, the homes of the state’s two major universities. Major universities perform research, and many of those research areas can be highly sensitive to vibration.
Prior to 2006, this area typically experienced three or fewer earthquakes per year, with an average recurrence interval of about 190 days. Starting in 2007, the number per year gradually increased. By 2015, the total exceeded 3300 events with magnitudes less than 4.2, a more than twenty-fold increase. This area covering only a fraction of Oklahoma has experienced a larger quantity of earthquakes than California and Nevada combined.
The quantitative difference from California or any other area with research facilities is a large part of their problem. The recurrence interval for micro-tremors can impact research requiring extended periods of quiescence for measurements or imaging. Though the microseismic activity is generally imperceptible, it represents a significant increase over ambient conditions. The instrument would experience no damage, but the data from the research might be contaminated. This paper presents measured data and explores the manner in which microtremors and short recurrence intervals might limit the research that can be carried out in central Oklahoma.
Introduction
Many areas of technology rely upon equipment or processes that are sensitive to vibration. The sensitivity is increasing as technology further develops. The most demanding areas include nanotechnology, semiconductor production, imaging (from micrometer to nanometer scale), medical research, and physics. In some instances, research organizations must provide low-vibration spaces in order to attract (or retain) highly-qualified researchers. Companies engaged in work with semiconductors, materials science, or pharmaceuticals may accept or reject a locale based upon the vibrations present there.
It is now standard practice to carry out a vibration survey at a location being considered for advanced technology. Likewise, environmental impact studies for systems or processes that could alter the vibration environment will assess whether those changes could affect existing or planned facilities. It was during a vibration study for a research facility in central Oklahoma that the authors became aware of how the changing seismicity there might adversely affect the work being planned at the proposed facility.
Vibration Sensitivity
Vibration sensitivity arises because some component of an instrument or experimental setup will respond in resonance (i.e., they will “ring”) when excited in some way. For instance, a vibration at a frequency near the resonance frequency can cause movement of a probe, or can cause misalignment of a beam, as is the case of an electron microscope or particle beam. Each instrument or process will have an inherent vibration threshold below which it will function properly, and above which it may provide erroneous data or undesirable performance. We call this a criterion—in this case, an instrument- or process-specific criterion.
A research facility may have several vibration-sensitive instruments, each with its own criterion, so it is typically more straightforward to work with a “generic” criterion, which will represent the collection of the criteria in that facility. A popular family of criteria—denoted the “VC” criteria, specified as VC-# where # is a letter—represents a wide range of vibration limits in terms of constant-amplitude spectra of rms velocity in one-third octave bands of frequency. [1] Stated in these terms, a generic laboratory on a suspended floor (i.e., supported on columns, with open space below) might typically be designed to meet VC-A, which has an amplitude of 50 µm/s.
Each increasing letter halves the previous amplitude, VC-B has an amplitude of 25 µm/s, VC-C is12.5 µm/s, etc. The criterion amplitudes and typical applications are shown in Table 1. Those who are more familiar with earthquakes might relate better to the human threshold of perception, which is 3 to 4 times VC-A (depending on the circumstances). The comparable threshold above which building damage might occur is on the order of 2500 µm/s, fifty times VC-A.
Another popular criterion is a hybrid spectrum developed for the design of NIST’s Advanced Measurement Laboratory in Maryland, and dubbed “NIST-A.” It has become the de facto criterion for a generation of nanotechnology buildings and is consistent with the published requirements of several types of instruments and processes. At frequencies above 20 Hz, it is identical to VC-E, and at lower frequencies it becomes more conservative as frequency decreases, with an amplitude value (expressed as rms vibration displacement amplitude rather than velocity) of 0.025 µm (or 25 nm). At 1.25 Hz, NIST-A and VC-I are numerically equal.
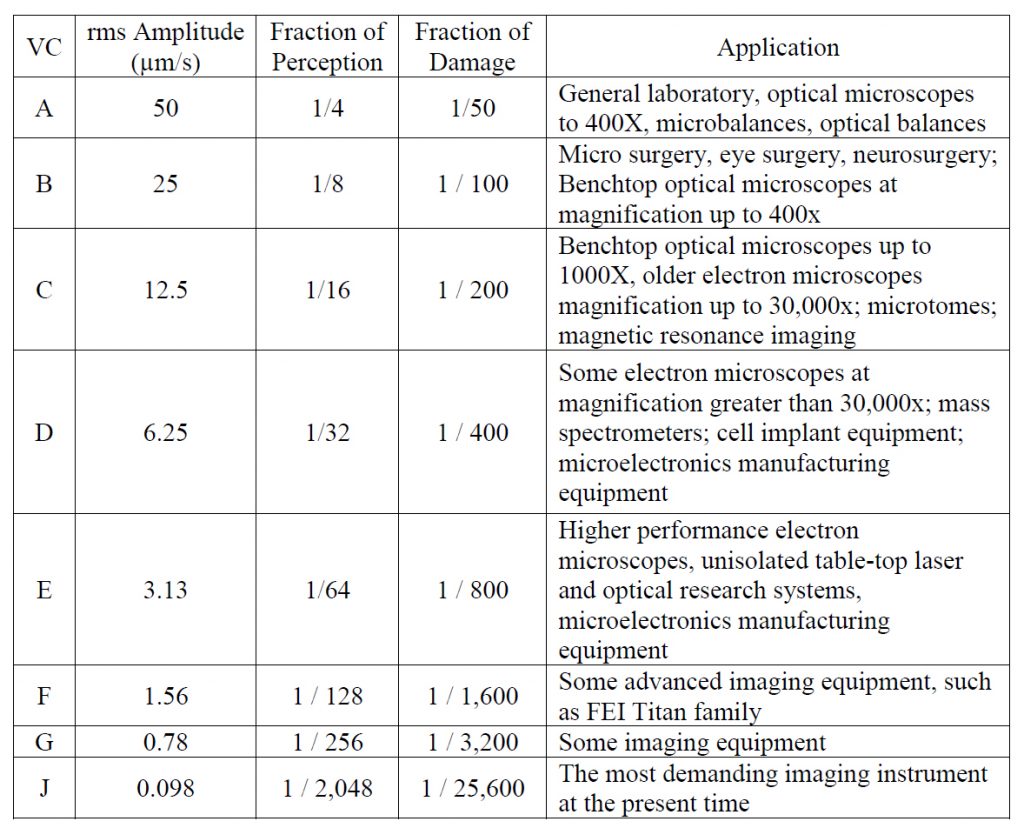
Vibration Site Survey
Experience has shown that the majority of what can be learned about the vibration environment can be obtained economically from a series of relatively short-term measurements (several minutes apiece) of ambient conditions at representative locations. Vibrations are measured in vertical and two orthogonal horizontal directions. This part of the study will capture the steady-state conditions (more appropriately called “stationary” conditions when random vibrations present), and additional spot measurements are used to document transient conditions such as vibration due to footfall and vehicle or rail traffic. When asked to perform a survey in Oklahoma, the researchers expressed a wish to know more about the vibrations associated with the increased seismicity due to oilfield activities. This suggested the use of a long-term monitoring protocol in which the vibrations were recorded digitally and post processed into statistical representation, along with selected spot measurements. [2]
Observations in Site Vibration Study
A vibration monitoring system was installed at a basement level in an existing building on the proposed site using three 10 V/g accelerometers in a triaxial configuration. The signal processing system was set to log one-third octave band energy-average spectra (Leq, with 60-sec averaging time), and operated for approximately 48 consecutive hours at the end of March 2015. Known disturbances of the system, such as when operators checked on the system or there was other known activity directly adjacent to the sensors, were removed from the data stream prior to statistical summary. Figure 1(a) shows the results of a statistical analysis of the horizontal north-south amplitudes over the full (but edited) monitoring period, in terms of centile spectra. [2] The spectra labeled “Max” and “Min” are the upper and lower bounds of the measurements. The other spectra, denoted “Ln”, are the spectra exceeded only n percent of the time, with leading zeroes added when n is less than 1.
We see that 90% of the time the vibrations meet NIST-A at frequencies above 4 Hz, but not at lower frequencies. The Max spectrum exceeds NIST-A at all frequencies below 16 Hz, and approaches VC-C at 1 Hz. Criterion VC-E is exceeded 5% of the time. Similar observations may be made regarding the east-west horizontal vibrations; the amplitudes of the individual vertical spectra are slightly higher than their horizontal counterpart.
Figure 1(b) shows a trace of the individual 1 Hz third-octave band 60-sec Leq values over the full 48-hr monitoring period. (The reason the lowest-frequency bands are important will become evident shortly.)
This trend is consistent throughout the monitoring period, as even the minimum amplitudes measured exceed the criterion at the lowest frequencies. The low frequency results also do not appear to match with the short-term spot measurement results from the same location (90-sec synthesized spectra using FFT with overlap), an example of which is shown in Figure 2. This measurement set met NIST-A in the low-frequency range that is problematic in Figure 1. Nominally, the presence of the low-frequency problem would suggest disturbance of the sensor group or close-proximity impacts on the floor. (Impulses can create a spectrum like what was observed in Figure 1.) However, the consistency of the low-frequency vibration implies a different source in this case.
The issue became clearer when a 120-sec segment of the recorded time signal was extracted from one of the more active periods (see Figure 1(b)) and examined in terms of the raw acceleration signal, shown in Figure 3. These impulse-decay patterns are suggestive of low-amplitude earthquakes (with peaks 0.004 to 0.006g), closely spaced in time. The short rise time at the start of each event will be interpreted by the spectral signal processor as an impulse, which has a spectrum spread over a wide frequency range. The individual spikes in the time trace in Figure 1(b) each correspond to a small-magnitude earthquake. The recurrence interval of even smaller magnitude micro-seismic activity suggested by Figure 3 is on the order of about 20 seconds or less. The known seismic activity in Oklahoma was of concern to the client, but was this the form it took?
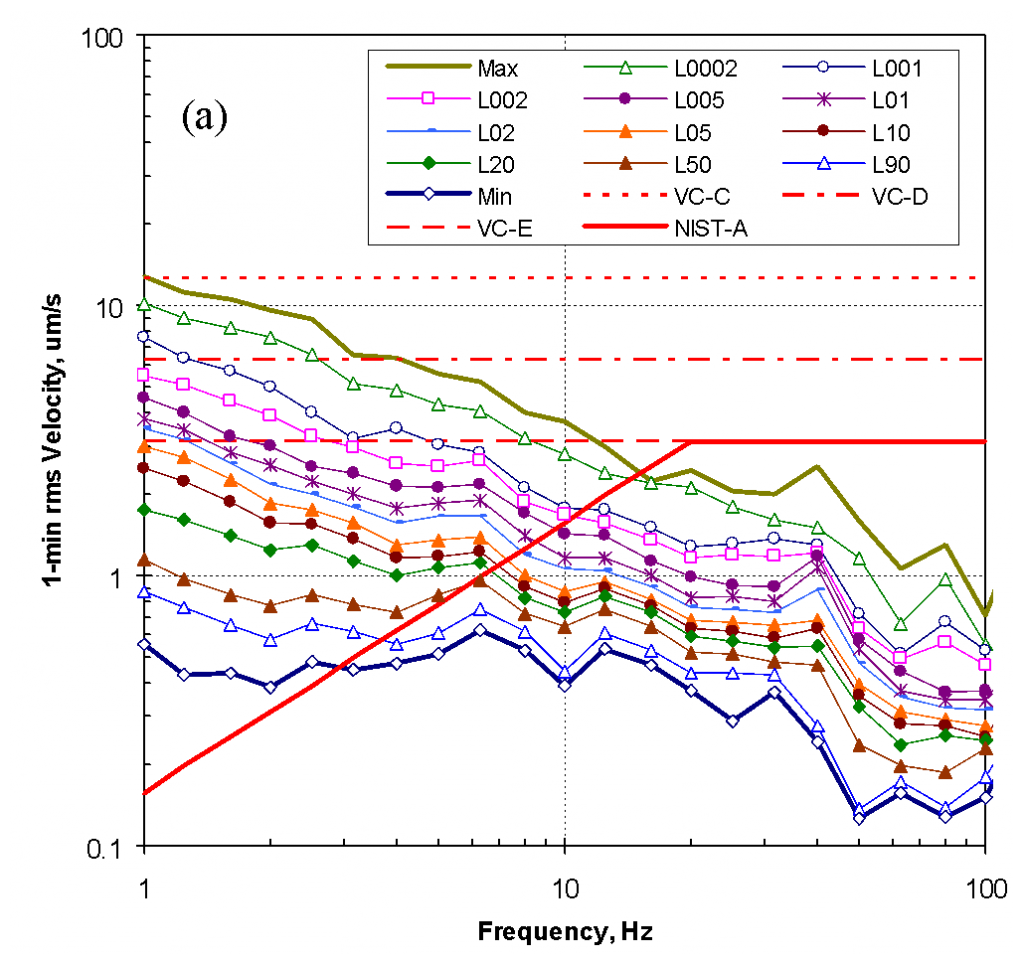
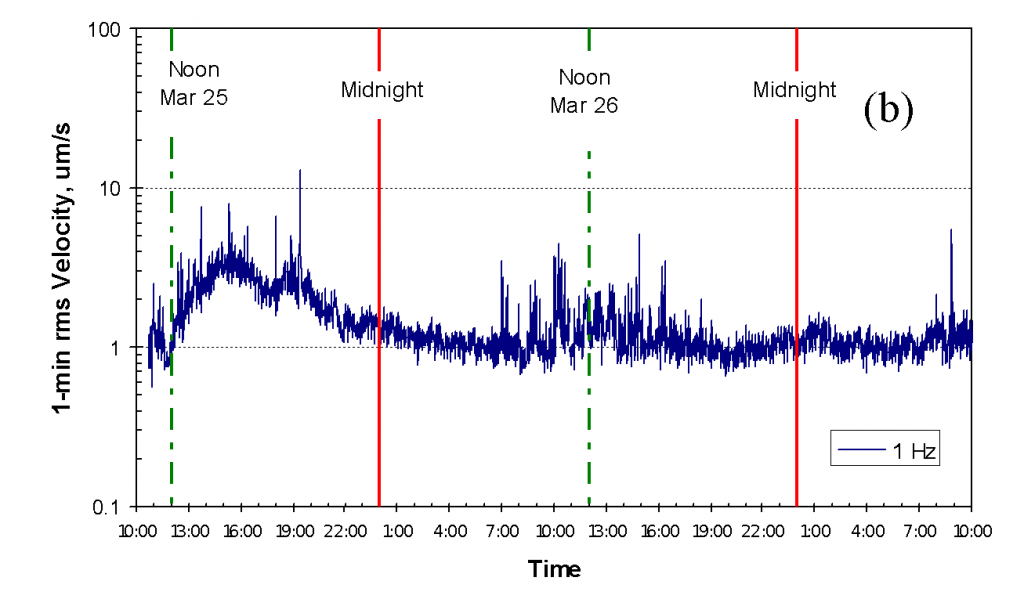
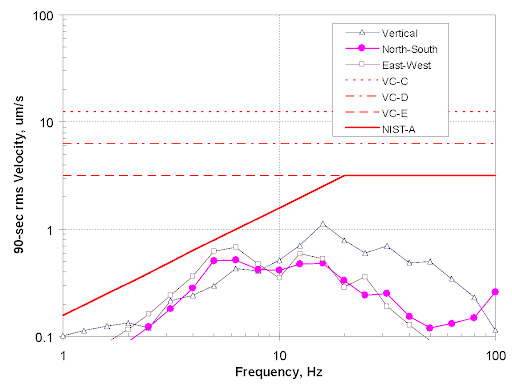
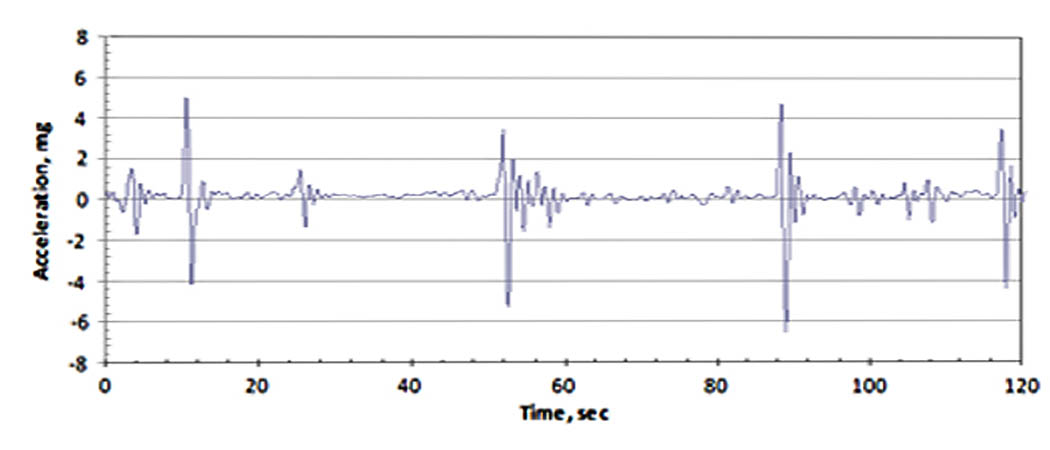
Figure 4 superimposes occurrence of earthquakes in the USGS archive for Oklahoma (using individual symbols) on Figure 1(a), allowing a direct comparison between the 1 Hz averages with individual events. In general, the time the vibration appears at the monitor location will lag behind the USGS-logged time because of travel time from the source. The maxima associated with three of these earthquakes are shown as spectra in Figure 5. (Typically, two separate spectra were observed which appear associated with P- and S-wave arrival. The general shape of these spectra is similar to the overall maxima, due to the short rise time discussed above.) The measured spectra during USGS-identified earthquakes were not the highest amplitudes measured during the monitoring period, and there does not appear to be a precise correlation between the time and number of logged earthquakes and spikes in our measured data. This implies that lower magnitude earthquakes, below what would have been logged by USGS, also have a significant impact.
Upon comparison of Figure 1(a) and Figure 2, one key difference is noteworthy. Figure 1(a) is an ensemble representation of both stationary and transient vibrations; and Figure 2 is a representation of a short duration deliberately taken between transient events. In site vibration evaluation, the stationary and transient vibrations are measured and reported separately. Usually, the transients—such events as train or vehicle passages—are well spaced in time, generally with enough time between transients to allow the necessary work to be carried out. The same thought process can be applied to the seismic events, using a process from seismology to assess recurrence intervals.
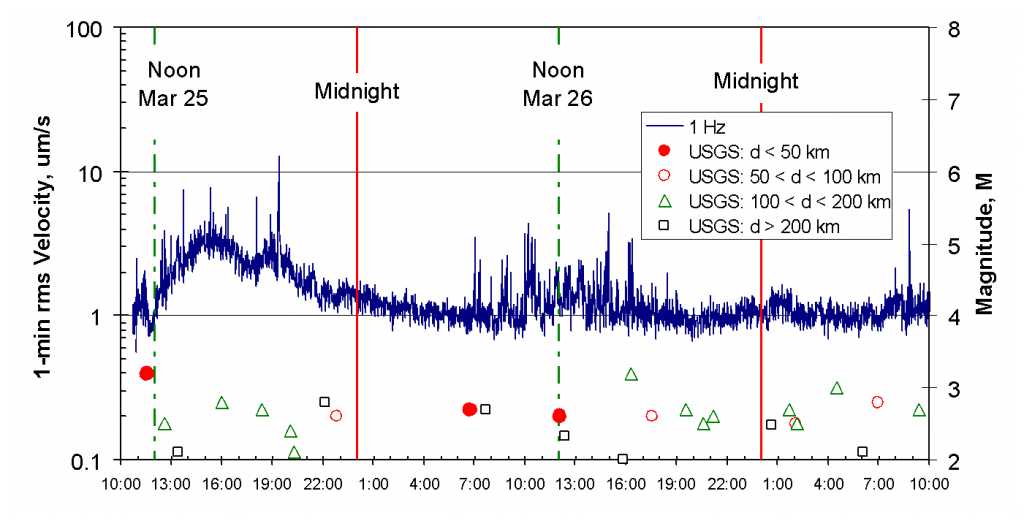
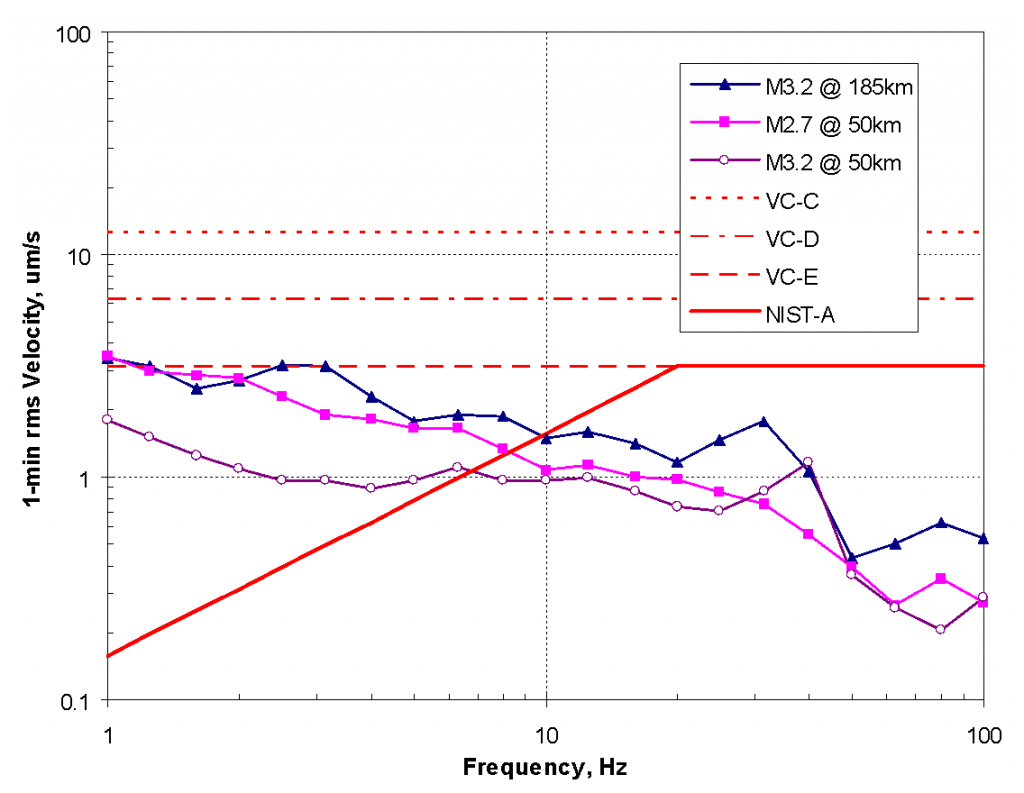
Examining the Seismic Setting
Since 2006, a swath of north-central Oklahoma has been plagued with a series of small earthquakes, most having magnitude less than 4.0. Figure 6 shows the location of earthquakes in central Oklahoma from 2010 through July 2015, taken from the database on the USGS site. According to the USGS earthquake archive, these events have been concentrated in a rectangular area centered along a line extending NNW from near McAlester and crossing Interstate 35 near Perry. The 130km x 260km (80 mi x 160 mi) rectangle’s long axis is along this line, and most of it lies north of Interstate 40, though one corner dips below I-40. Norman is near the edge of the rectangle; Stillwater lies north of Norman and is well within the rectangle. These two cities are the homes of the state’s two major universities.
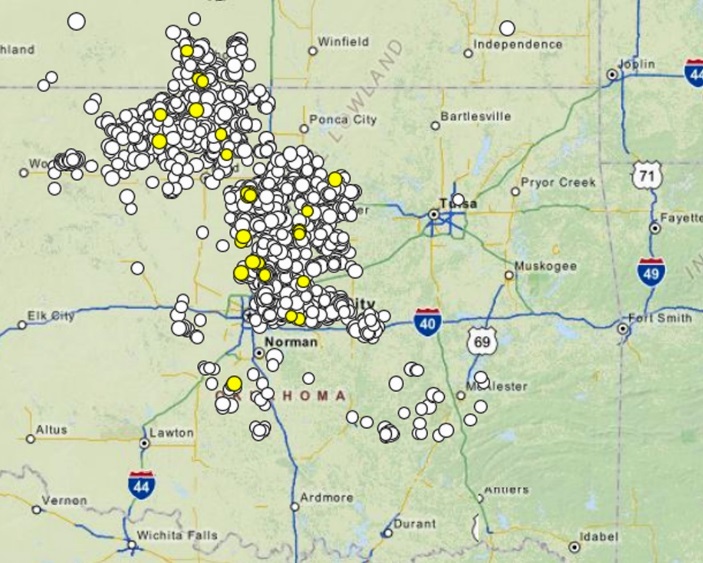
Prior to 2006, this area typically experienced three or fewer earthquakes per year, with an average recurrence interval of about 190 days. By comparison, over the two decades prior to 2006, the number of earthquakes in the US with Richter magnitude greater than 2.5 ranged from about 700 to over 2,000 (averaging about 1000), the majority occurring along the West Coast and Nevada. The northern Rocky Mountain states experienced between 40 and 330 earthquakes per year, averaging about 90 per year. In this 20-year period, the largest Oklahoma earthquake had a magnitude of 4.2.
Starting in 2007, USGS data show a generally increasing number of earthquakes per year in Oklahoma. Between 2010 and 2012, there were between 80 and 130 earthquakes per year, and the accelerating trend continued into 2013 and beyond. In 2014 the total was close to 2000, and since then, this area covering only a fraction of Oklahoma and a small part of southern Kansas has experienced a larger quantity of earthquakes than California and Nevada combined. Oklahoma’s total in 2015, the year of the measurements presented here, was approximately 3400 events with magnitudes less than 4.2. Most of the Oklahoma events reported by USGS are for M ≥ 2.5, but a small fraction—with epicenters in southern Kansas—are 2 ≤ M ≤ 2.5. This is simply a difference in the reporting protocol; seismology dictates the presence of events of even smaller magnitude, which occur even more frequently.
Seismicity involves random events which are traditionally represented statistically, in terms of Richter magnitude versus frequency of occurrence (events per unit time). The most commonly used seismic recurrence model is the Gutenberg-Richter Law, stated as
log λm – bM
(1)
where λm is the mean annual rate of exceedance of magnitude M, 10a is the mean yearly number of earthquakes of magnitude greater than or equal to zero (i.e., M ≥ 0), and b describes the relative likelihood of earthquakes. Youngs and Coppersmith provided an implementation of Gutenberg-Richter that is commonly used to address seismic hazard assessments over a wide range of magnitudes. It makes a distinction between seismicity and geologic effects. A threshold magnitude separates the seismic behavior (at lower M) from geological behavior). [3] The recurrence interval for earthquakes—assuming they are distributed randomly over time—can be obtained by dividing the period during which the events are counted by the number of events.
Figure 7 illustrates the fitting of Gutenberg-Richter for four sampling periods in Oklahoma and a representative one for the Bay Area. The symbols represent data from the USGS archive, and the dashed lines extrapolate the slope to lower magnitudes. The topmost curve is for the quarter in which the preceding measurements were made. The gray arrow indicates where the extrapolated curve crosses 1×106 events at M of approximately 0.5. This corresponds to a recurrence interval of about 32 seconds, on the order of what was observed above. The events represented in the preceding measurements most likely have magnitudes between M=0 and M=1. Additional study would be required to develop a correlation between magnitude, distance, and microvibration at the site.
Figure 8 provides a means of comparing the relative seismicity of Oklahoma and the Bay Area in terms of recurrence intervals for M=0.5 events. The recurrence interval for the Bay Area is almost constant, between 40 and 100 minutes. Prior to 2010, the recurrence interval for Oklahoma was greater than that of the Bay Area (and was on the order of 70 days between events prior to 1999), but in 2014 the recurrence interval dropped below that of the Bay Area, where it continues. The gray arrow indicates the quarter in which the previous measurements were made. The situation has improved, and in Q3 2017, the recurrence interval was about 10 minutes.
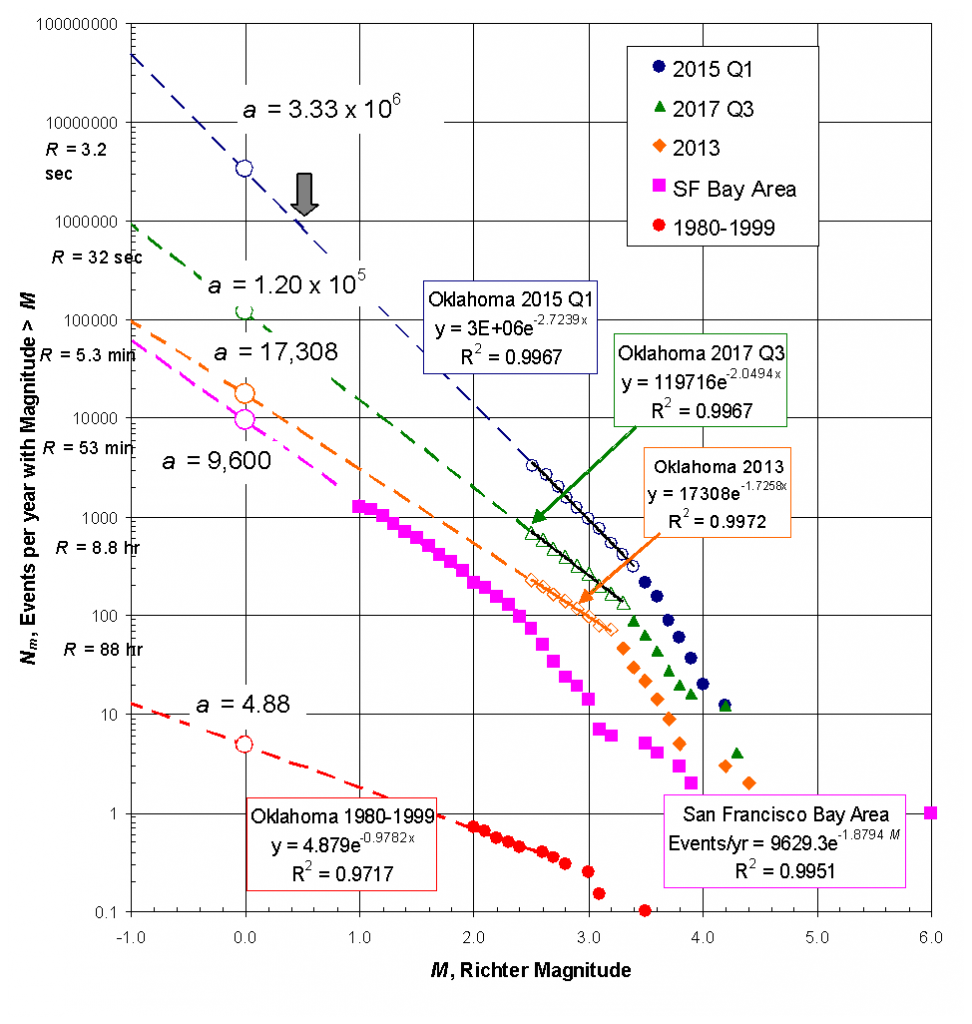
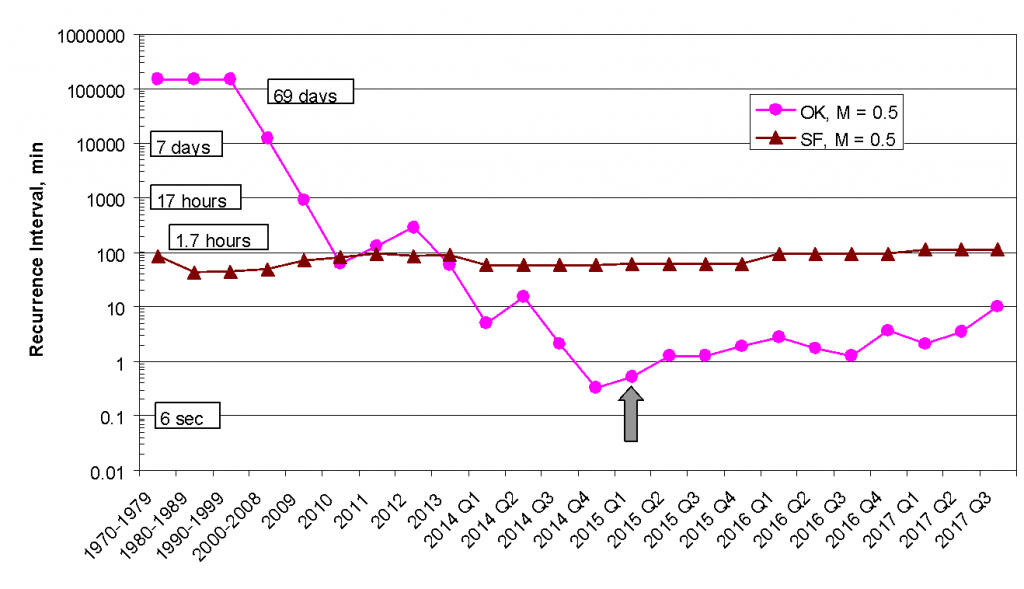
Conclusion
A detailed vibration survey for a research facility in central Oklahoma suggests that in addition to vibration impact from stationary ambient conditions and known transients such as rail and traffic, an assessment must consider recurrence intervals between low-amplitude seismic events. This study indicates that individual events that would be disruptive to vibration-sensitive work may be associated with microseismic events with magnitudes between -1 and 1, which are not even reported in the USGS archive. (Like decibels, the Richter magnitude scale can go to zero or into negative numbers.) There are also discrete earthquakes of magnitude greater than 2.5.
The highest maximum-hold amplitude we observed was on the order of VC-C at 1 Hz and below VC-D at frequencies above 4 Hz. It dropped below VC-E and NIST-A near 10 Hz. This site meets NIST-A between seismic events, but the typical microseismic activity results in spectra lying above NIST-A but below VC-D.
The low frequency impacts will not be apparent in spot measurements if they are taken on the basis of rejecting what appears to the experienced operator to be spurious noise. If a measurement is deliberately placed in a “quiet” period between events, it gives and accurate assessment of what is possible for very short periods, but not for long-term measurements, observations, or experiments. Vibration isolation systems exist which can deal with this excitation, and their justification must be based on acceptability of estimated recurrence intervals, not actual amplitudes.
In the case discussed here, the client decided on a “wait-and-see” approach. The facility is under construction and once it is completed, the researchers will estimate whether the intermittent seismic vibrations are problematic for their work. They may require vibration attenuation on the order of 50-60 dB at 0.8 Hz in order to meet the NIST-A criterion for extended periods. This will involve a rather exotic hybrid vibration isolation system. It is possible, but it won’t be inexpensive.
The data suggest that there are still microtremors at night, but they are much smaller than during the day. One hypothesis, unconfirmed at this time, is that if the seismicity is due to wastewater injection, as seems to be a prevailing opinion (though the press often uses the word “fracking”), then it may be the case that injection is carried out during the day (causing larger events) and not at night. At night, the ground may be adjusting to the new deformation state following the larger events, in much the same manner (and reason) as aftershocks following a significant earthquake.
Clearly, however, a site vibration assessment of a site for advanced technology must include an examination of the local seismicity if the vibration-sensitive processes require extended periods of quietude. Because generation mechanisms and wave propagation properties are likely site dependent, the evaluation should include an extended monitoring period and an extrapolation of seismicity using the USGS archive.
References
1. H. Amick, M. Gendreau, T. Busch, and C. Gordon, “Evolving criteria for research facilities: vibration,” Proceedings of SPIE Conference 5933: Buildings for Nanoscale Research and Beyond, San Diego, CA, 31 Jul 2005 to 1 Aug 2005
2. H. Amick, M. Gendreau, & N. Wongprasert, “Centile spectra, measurement times, and statistics of ground vibration,” Proceedings of the Second International Symposium on Environmental Vibrations: Prediction, Monitoring, Mitigation and Evaluation (ISEV2005), Okayama University, Okayama, Japan (20 to 22 September 2005).
3. Steven L. Kramer, Geotechnical Earthquake Engineering, Prentice-Hall, 1996.
HA-SL_11NCEE_Okla-EQsReprinted from Proceedings 11th US National Conference on Earthquake Engineering, Los Angeles, California, 25-29 June 2018.